Letter to Editor
Toward Tresino phase-transition Power
Mayer Applied Research Inc., USA
*Corresponding author: Frederick J. Mayer, Mayer Applied Research Inc. 1417 Dicken Drive, Ann Arbor, MI 48103, USA, E-mail: fred@frederickjmayer.net
Received: November 17, 2023 Accepted: November 28, 2023 Published: December 8, 2023
Citation: Mayer FJ. Toward Tresino phasetransition Power. Int J Cosmol Astron Astrophys. 2023; 5(2): 237-239. doi: 10.18689/ijcaa-1000144
Copyright: © 2023 The Author(s). This work is licensed under a Creative Commons Attribution 4.0 International License, whichpermits unrestricted use, distribution, and reproduction in any medium, provided the original work is properly cited.
Abstract
Recent studies of solar coronal-heating and solar-wind generation suggests a program to achieve a laboratory demonstration of the solar process should be undertaken.
Keywords: Tresinos, coronal-heating, solar-wind
Introduction
Most, but not all, of the energy generated by the Sun originates from nuclear fusion at the solar core. Attempts to achieve similar fusion energy releases in a laboratory setting has proven to be challenging due to the extreme plasma conditions (high temperatures) required. On the other hand, the solar energy observed in the coronal heating and solar wind, that have been the focus of my recent research, has determined that the plasma conditions near the solar edge appear to be considerably less challenging to achieve in the laboratory.
In my recent Letter [1] the data regarding the solar-power produced at near the solar surface by the tresino phase- transition was determined and furthermore a previous Letter [2] found similar plasma characteristics beneath the solar surface. So, the present Letter presents initial thoughts on how the tresino phase-transition might be accessed in the laboratory and perhaps even as a power source. There are important reasons why this possibility should be considered: (1) the new energy source is non-nuclear as well as non-radioactive, furthermore, (2) the exhaust gases are not toxic. Of course, all of this will be only speculation until laboratory experiments can be produced that show that such reactions can be achieved.
In Letter [1] the important plasma data were the density ne ≈ 5 × 1010 / cm3 and the temperature Te≈17.5 eV close to where the tresino phase-transition begins. After the plasma has expanded, the density drops down to ≈1.6 × 109 / cm3 where the tresino phase-transition begins to release the new energy as both heat and ion-flux like the solar-wind. Note that the starting plasma conditions compared to those of the solar core are quite modest and may be achievable with a number of pulsed-power sources such as lasers, electron beams, and/or microwave sources. Because the plasma conditions required are most important, a brief review of the dynamics of the required tresino phase-transition is given in the review.
A Simple Example
Figure 1 is an example of a laser-driven laboratory experiment. A low-density hydrogen gas is allowed to flow through a small (ceramic) funnel-shaped pinhole into a vacuum chamber such that the density increases in the throat of the pinhole. Then at the appropriate moment a laser-heating pulse is directed into the now-compressed gas igniting a plasma slab which is allowed to expand outward through the diverging aperture. Magnetic-field pick-up coils will be placed around the resulting plasma-outflow and used as a diagnostic tool. Perhaps this experiment can demonstrate the tresino phase-transition just like the solar-edge in [1].
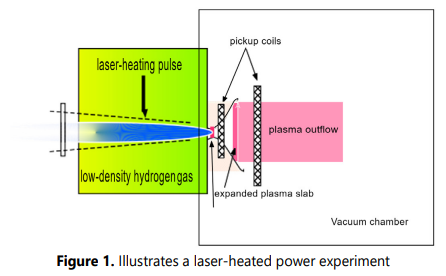
Other pulsed-power systems may be more successful helping to select the system for further development, especially toward higher energy releases. An example is to make use of spherical targets in the laser illumination system as shown in Figure 2 below. This system was designed and used by our early KMS Fusion group [3] in irradiating small glass shells (100 micron) containing DT gas but would be suitable for irradiating thin plastic shells at the (centimeter scale) containing hydrogen gas. In this case, the laser light penetrates the plastic shells to ignite the hydrogen plasma. This laser-target illumination system would make diagnostic access easier through the annular gap. Perhaps this laser ignition system could be advantageous for scaling-up the energy release.
Review of the Tresino Phase-Transition Dynamics
It is useful to begin this review by writing down some parameters of the phase-transition plasma. The Debye length is 0.078 cm; the number of electrons in this Debye sphere is 3.14×106; the mean electron-electron distance is 0.00085 cm; the electron thermal velocity is 1.7 × 108cm/s and the mean-time between electron collisions is 4.9 × 10−12 seconds.
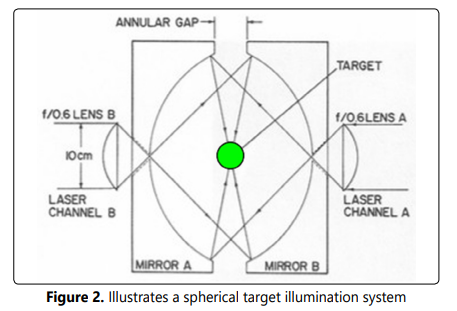
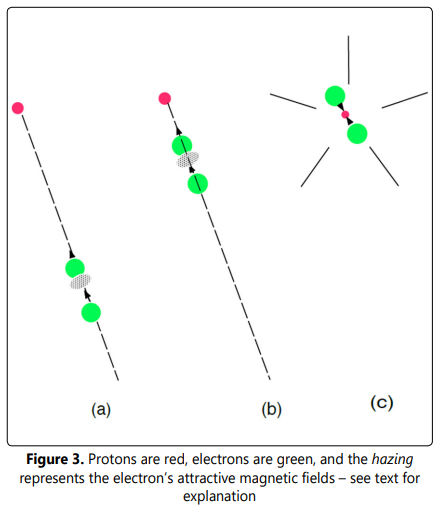
Now let’s examine the tresino phase-transition process by which the centrally-located proton in its Debye sphere combines with a tandem-electron pair to form a tresino. Figure 3 illustrates the temporal sequence of the phasetransition, initiated by the formation of a tandem-electron pair previously derived in [4]. In Figure 3(a), the tandem- electron pair forms when polarized in the direction of the electric-field of the proton, which then accelerates toward the proton while the two electrons are pulled together further in Figure 3(b) finally reaching the tresino’s potential- well resulting in the creation of the new tresino depicted in Figure 3(c). Because the tresino is a stable bound state composite, it releases its formation energy (≈ 3.7 keV) when created.
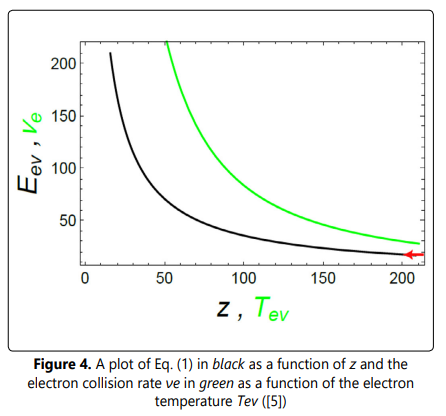
In the derivation of [4], I showed that the dynamics of the tandem-electron pair could be written as:
Ec[(−1/(2z3) + (1/z))] Eq (1)
where Ec≈ 3.7 keV and z = d/λc is the distance between the tandem-electrons, measured in Comptons. Figure 4 plots Eq (1) in black as a function of z, as well as the electron collision rate νc ([5]) in green as a function of the electron temperature Tev.
It is important to notice that the tandem-electron pair originates at close to the solar (baseline) temperature, 17.5 eV, (note the red arrow in Figure 4) and accelerates rapidly toward the proton centered in its Debye sphere. This happens because it is the only doubly-negative charged object in the protons field of view and it is therefore strongly attracted to the proton. Note that such an electron pair cannot form further up the path to the proton because of the increased collision rate at the higher temperatures, see Figure 4. Hence, multiple electron collisions interfere with the formation of the pairs at higher-temperatures.
Closing Remarks
The observations regarding explosive solar-energy releases have been traced to the tresino phase-transition in my recent Letters. If this physics is in fact correct, it suggests laboratory exploration of this possibility should be undertaken. Initial recommendations regarding laboratory experiments have been suggested herein but additional approaches may be considered in the future.
References
- Mayer FJ. How the Tresino Phase-Transition Heats the Solar Corona and Energizes the Solar Wind. Int J Cosmol Astron Astrophys. 2023; 5(1): 208-209. doi: 10.18689/ijcaa-1000139
- Mayer FJ. The Phase-Transition Beneath the Solar Surface. Int J Cosmol Astron Astrophys. 2021; 3(1): 121-124. doi: 10.18689/ijcaa-1000125
- Mayer FJ. Spin-Mediated Electron Pairing. Int J Phys Stud Res. 2021; 4(1): 95-97. doi: 10.18689/ijpsr-1000115