Research Article
Initiating Tresino Phase-Transitions in Laboratory Hydrogen-Plasmas
Mayer Applied Research Inc., USA
*Corresponding author: Frederick J. Mayer, Mayer Applied Research Inc. 1417 Dicken Drive, Ann Arbor, MI 48103, USA, E-mail: fred@frederickjmayer.net
Received: November 07, 2022 Accepted: November 23, 2022 Published: December 17, 2022
Citation: Mayer FJ. Initiating Tresino PhaseTransitions in Laboratory Hydrogen-Plasmas. Int J Cosmol Astron Astrophys. 2022; 4(2): 199-201. doi: 10.18689/ijcaa-1000136
Copyright: © 2022 The Author(s). This work is licensed under a Creative Commons Attribution 4.0 International License, which permits unrestricted use, distribution, and reproduction in any medium, provided the original work is properly cited.
Abstract
This paper introduces a recently-recognized hydrogen-plasma energy release process and examines ways to initiate the process in laboratory-based hydrogenplasmas. In addition, the process has possible implications in present hydrogen-plasma experiments showing the importance of tresino physics.
Keywords: Tresinos, coronal mass ejections
Introduction
In the early decades of last century, much was learned about atomic physics and quantum mechanics from telescopic observations of the Sun [1]. In the 1930s, Hans Bethe and his colleagues [2] determined that nuclear reactions under the enormous pressures in the solar core were responsible for the energy production in the Sun. Moreover, the reactions were determined to be a group of fusion interactions. Importantly, these new understandings later gave rise to a number of designs for fusion-power reactors that were hoped to be the successors to fission reactors. Unfortunately, attempts to show that fusion reactors represented a viable power system have not yet been demonstrated but they continue under development around the world.
However, there is another aspect of solar observations that have yet to be fully understood; specifically, the gigantic explosions often referred to as “coronal mass ejections” or CMEs. These very large explosive events cannot be fusion reactions like those in the solar-core because they originate at plasma densities much too low compared to those of the core. Nonetheless, the observations are important because they appear to be a fundamental source of hydrogen-plasma energy generation, the focus of my recent CME research.
Tresino Physics
Readers unfamiliar with tresinos will benefit from reading the Introduction section in my paper on “Hidden Baryons” [3], as tresinos are crucially important to understanding how the hydrogen-plasma energy source obtains. This paper includes a basic (classical) derivation of the tresino composite system. After becoming comfortable with what tresinos are, it might be further useful to understand their impact in geophysics [4] and cosmology [5], before I discuss their importance in the Sun. Many of the papers cowritten with my late colleague (John R. Reitz) and me over the past years about tresinophysics, can be found in the just-cited references.
The Tresino Phase-Transition in the Sun
As John and I concluded in our “Early Universe” paper [5], it was important to determine if there was a location in the Sun that supported the tresino phase-transition. In my recent paper [6], I found that indeed there was such a location at a somewhat surprising depth of only 2350 km below the solar surface. This location was found between lines n = 26 through n = 29 of Avrett & Loeserʼs Model C7 of the quiet Sun. The average plasma densities and temperatures from these data were found in [7], to be:
ne = 1.6 109/cm3 and T = 17.5 eV.
These parameters represent the conditions expected to produce the energy release in laboratory hydrogen-plasmas as well. I note here, also from [7], that a one cm3 sample at the phase-transition conditions would yield about 5.9 MeV to the local environment; with, of course, a smaller energy-release expected from smaller samples.
In addition, this latest paper [7] focussed on the microphysics of how the tresino phase-transition actually takes place. Interestingly, Debye spheres play an important role in how this happens. Note that Debye spheres insulate centrally-located protons from the surrounding electrons in an electrostatic-equilibrium. When the Debye spheres are destabilized, a “flood” of electrons flows into the vicinity of the protons; after which protons may capture two electrons converting them into tresinos. When this happens, the tresinos “binding energy” is released into the local plasma environment.
Tresino Phase-Transitions in Laboratory Plasmas
It is expected that laboratory-experiments observing the tresino phase-transition will generally be of two types: Those by design and those by “accident”. In the first case, there can be many possibilities depending upon a laboratoryʼs source of energy deposition. One such example is presented in Figure 1 below where a laser-heating pulse is focussed into the throat of a pinhole aperture through which hydrogen gas has been allowed to flow into a vacuum chamber. The laser wave-length and pulse-length will have to be adjusted along with the gas flow rate to achieve access to the tresino phase-transition temperature and the phase-transition density. When successful, the phase-transition releases the tresinos “binding energy” as streams of tresinos and protons and their associated magnetic fields. Among other detection methods, the exhaust streams can be monitored by pick-up coils surrounding the exiting “plume”. In the second case, the phase-transition by “accident”, it is possible that the tresino phase-transition has already been “accidentally” encountered in laboratory hydrogen-plasmas but has not been recognized as such, except that some extra energy may have been observed.
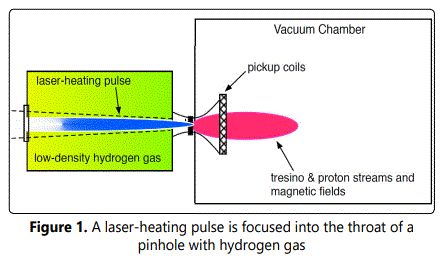
This is perhaps most likely and may have happened in plasma-pinches such as plasma focus devices [8], small Z-pinches [9], and/or large Z-pinches [10]. In some experiments, deuterium gas may have been used, in which case deuterontresinos would have been created, which in turn would have allowed them to undergo d*-d nuclear reactions (where d* represents a deuteron-tresino). Such tresino-driven nuclear reactions have already been discussed in [11] where the electron screening of the deuteron-tresino plays a significant role in enhancing the reaction rate of the otherwise wellknown d-d nuclear reactions due to the substantially-reduced Coulomb barrier repulsion.
Finally, another example of “accidental” tresino formation might occur in a force-balanced plasma configuration (e.g., tokamaks) wherein a tresino phase-transition zone forms (accidentally) producing excess energy but also disrupting the force-balance, clearly a net-negative result in such an experiment.
Final Remarks
Experiments that access the tresino phase-transition in a laboratory hydrogen-based plasma are important because they could confirm the nature of CMEs on the Sun and could also provide additional basic plasma-physics understanding. Variations of the nuclear reactions noted above might also yield benefits in the development of fusion reactors. For example, if a design of an experiment can be found that maintains the phase-transition plasma conditions for an extended time, it could offer a new method for producing a large-scale hydrogen-energy system either with or without nuclear reactions.
Accessing the tresino phase-transition could also provide an approach to initiating tresino-driven nuclear reactions such as 6 Li(d*, p) 7 Li+5 MeV and 11B(p*, α α)α+8.7 MeV. These reactions may be accelerated due to the previously-mentioned tresino interactions having lowered the Coulomb-barrier as discussed in [11]. Finally, experiments using gas mixtures, e.g., deuterium and tritium, might also be useful in some special situations in ongoing fusion programs. It is my hope that laboratory experiments like these can be fielded in the near future and are found to be successful.
Dedication
I dedicate this paper to my late mentor, collaborator, and friend, Dr. John R. Reitz, without whose efforts, this work would not have been possible.
References
- James McAteer. Solar Observations throughout History. New Mexico State University. 2004.
- Landmarks: What Makes the Stars Shine. Phys Rev Focus. 2008; 21: 3.
- Mayer FJ. Hidden baryons: The physics of Compton composites. EPL. 2016; 114: 69001-69007. doi: 10.1209/0295- 5075/114/69001
- Mayer FJ. Geophysical Implications of Tresino Formation: A Narrated Review. Int J Earth Sci Geol. 2020; 2(1): 86-89. doi: 10.18689/ijeg-1000110
- Frederick Mayer, John Reitz. Compton Composites Late in the Early Universe. Galaxies. 2014; 2: 382-409. doi:10.3390/galaxies2030382
- Mayer FJ. The Phase-Transition Beneath the Solar Surface. Int J Cosmol Astron Astrophys. 2021; 3(1): 121-124. doi: 10.18689/ijcaa-1000125
- Mayer FJ. The Physics of the Tresino Phase-Transition beneath the Solar Surface. Int J Cosmol Astron Astrophys. 2022; 4(1): 158-160. doi: 10.18689/ijcaa-1000129
- Dense Plasma Focus, Plasma-Universe.com
- U. Shumlak. Z-pinch fusion. J Appl Phys. 2020; 127: 200901. doi: 10.1063/5.0004228
- DB Sinars, MA Sweeney, CS Alexander, DJ Ampleford, T Ao, JP Apruzese, et al. Review of pulsed power-driven high energy density physics research on Z at Sandia. Physics of Plasmas. 2020; 27: 070501. doi: 10.1063/5.0007476
- Mayer FJ. Reitz JR. Thermal energy generation in the earth. Nonlin Processes in Geophys. 2014; 21: 367-378. doi:10.5194/npg-21-367- 2014