Mini Review Article
Geophysical Implications of Tresino Formation: A Narrated Review
Mayer Applied Research Inc., Ann Arbor, MI 48103, USA
*Corresponding author: Frederick J Mayer, Mayer Applied Research Inc. 1417 Dicken Drive, Ann Arbor, MI 48103, USA, E-mail: fmayer@sysmatrix.net
Received: November 18, 2020 Accepted: December 02, 2020 Published: December 11, 2020
Citation: Mayer FJ. Geophysical Implications of Tresino Formation: A Narrated Review. Int J Earth Sci Geol. 2020; 2(1): 86-89. doi: 10.18689/ijeg-1000110
Copyright: © 2020 The Author(s). This work is licensed under a Creative Commons Attribution 4.0 International License, which permits unrestricted use, distribution, and reproduction in any medium, provided the original work is properly cited.
Abstract
Recent understandings pertaining to tresinos in laboratory experiments and to geophysical observations represents a new paradigm for Earthʼs energy generation as well as an exciting new direction toward developing tresino generated power reactors.
Keywords: Geophysical implications; Tresino formation; Cold-fusion; Low-energy nuclear reactions.
History and Introduction
This paper reviews the physics and geophysics results of my late colleague, John Reitz, and me over the past number of years; it is presented in the form of a physics narrative, in part because all our work has been previously published. The narrative form avoids duplication but importantly shows how the various results of our work over these years are interconnected; the mathematical details and physics/geophysics arguments may be found in our referenced publications. I hope this presentation will make the importance of our work easily understood, retrieved, and useful.
Our efforts started with the research into the area initially called cold fusion and later referred to as low-energy nuclear reactions. Having had substantial experience in nuclear physics these experiments clearly presented a challenge to contemporary physics as we explained in our IJTP paper [1]. Therefore, we decided to examine possible alternative particle composites that may have been overlooked in the early days of the development of nuclear and atomic physics in the last century. After considerable efforts along these lines, including numerous false starts, we finally came to focus on a new conceptual configuration - an apparently strange Compton-scale composite, specifically the tresino (shown schematically in figure 1) that might be responsible for the experimental observations. Indeed, observations in other areas of physics were also suggested in this early paper and have been discussed in other publications.
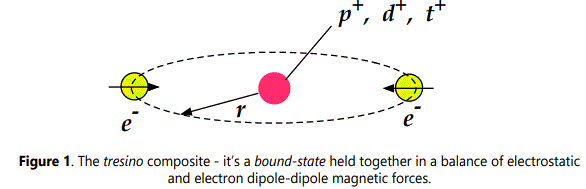
To many readers, the tresino may appear strange because it has a net-negative charge; how a proton acquires its two electrons in the tresino is both interesting and complicated as I discuss in the sections “Superconductivity and Cold Fusion and Superconductivity and Energy Generation in Geophysics”. Importantly, the tresino is a bound-state (of ≈3.7 keV) so when itʼs formed it must release its binding-energy; furthermore it will persist unless the binding-energy is somehow resupplied. Note that a second proton neutralizes the proton tresino at atomic mass two.
Although our basic picture from this IJTP paper did have implications for the cold fusion issue (see Superconductivity and Cold Fusion section), we considered that the somewhat less controversial research involving the energy released from the Earth might be a better early application of tresino-formation physics; so we proceeded with our research in the geophysics arena. (Note: The nominal depth at which the energy generation in the Earth obtains is discussed in the Heat-Flow from the Earth section).
Tresinos and Energy release from the Earth
We had been aware that there were numerous problems for decades within the existing geophysics data and we discussed these in our paper [2]. After reviewing these issues, we developed the tresino-based physics that we then showed could resolve many of these problems. In particular, we showed how this physics correctly gave rise to the ratios of 3He and 4He over decades after their generation from the formation of: 1) proton tresinos, and the later-arising deuteron-tresino nuclear reaction chain. The integration of the reaction rate equations resulted in plots of the various species as functions of time. The deuteron-tresino nuclear reaction chain gave rise to the origin of 3He, to energy generation, and furthermore, the ratio of 3He and 4He. Figure 2 shows this ratio. As our paper showed, this ratio agrees well with the geophysical data that observed this ratio is orders of magnitude higher early in time (or closer to the reaction zone) and is ≈10-5 decades later (or much farther from the reaction zone). Perhaps more interesting, due to the energetic 4He at the end of the deuteron nuclear reaction chain some secondary nuclear reactions were found (in our atmosphere) for the otherwise difficult to explain but experimentally observed excess nuclides such as 10Ne and 40A.
Although this paper did show that tresinos could generate the low-energy nuclear reactions, at that time we did not understand the physics of how the tresinos acquired their electron pairs; this physics is discussed in the sections “Superconductivity and Cold Fusion and Superconductivity and Energy Generation in Geophysics”.
Magnetotellurics
Here the discussion begins with my attempts to more fully understand the physics of magnetotelluric (MT) images. Letʼs examine one such example presented in figure 3. I started by examining Chapter 3 by Professor Rob L. Evans in [The Magnetotelluric Method: Theory and Practice] [3]. It seemed clear to me that there was considerable uncertainty regarding the physical mechanisms that produce certain highly-conductive regions around the Earth. As this was the case, I had suggested [4] that the mechanism overlooked in the theory of the magnetotelluric surveys is that of superconductivity in certain Earth-based materials at special locations. In his discussion of the mechanisms, Evans has a section (page 76) on carbon as an ofteninvoked source of the high-conductivity zones but he finds it to be generally not too compelling, hence inconclusive. I point out that Professor Evans did not consider that, in some laboratory experiments in recent years, have found some carbon compositions display a marked superconductivity [5]. Although this latter paper is suggestive, a more directly relevant series of recent experiments [6] has shown superconductivity in processed granular carbon (powder) processed with added water and heating to produce superconductivity at elevated temperatures. This suggests a specific mechanism that would be accessible to much of the available carbon, in some form, found in the relatively near- surface geologic formations in the Earth. Of course some other materials might produce this effect but the Scheike et al., experiments appear to be a basis for further examination for understanding both the high-conductivity MT images. Furthermore they may also be required for the thermal energy generation in the Earth [2] by delivering electron pairs in tresino-formation.
Superconductivity and Cold Fusion
Recently, I had become aware [7] of an earlier published paper regarding an experiment in cold fusion that revealed high-loading of hydronium ions (H3O+) into a palladium cathode induced a superconducting phase transition in the electron fluid, i.e., that created Cooper pairs, along with some energy release from the formation of tresinos. Figure 4, copied from this paper, shows how the Cooper pairs combine with the hydronium ions to generate the energy release in tresino formation. This was an important observation that showed how superconductivity (Cooper pairs) in laboratory experiments had allowed the generation of energy from the formation of tresinos, hence this answered the question “how did tresinos acquire their electron pairs?” Perhaps most important, this physics was required to release the tresino-formation energy.
Superconductivity and Energy Generation in Geophysics
Even though tresino generation in cold fusion appears in a laboratory situation because Cooper pairs are being formed at high-loading of hydronium ions in palladium cathodes, there would be no such generation in the Earth. So what could be happening in the latter situation? The answer can be found by noting the above mentioned observations regarding magnetotellurics and examining my recent papers [4,7]. In geophysics some regions are found that have a ready supply of Cooper pairs. This might be expected because carbon is the 15th most abundant element in the Earthʼs crust and in some places it is not combined with other elements in minerals. As shown in the studies by Yankowitz et al. and Scheike et al. [5,6], carbon, probably in the form of graphite powder, is present to provide for the Cooper pairs resulting in the magnetotelluric images and with sufficient water (i.e., hydronium ions) present to create the formation of tresino-formation energy release. So, in the geophysics situation, the combined availability of carbon (with its Cooper pairs) along with the presence of enough hydronium ions (enough water) the release of energy then starts the tresino-formation energy transition. Although isolated carbon deposits may be likely, carbon in carbonatites [9] with multiple carbon surfaces or interfaces, represent another possibility.


Heat-Flow from the Earth
In our early work on the energy generation in the Earth [2] we gave an estimate of where the energy was being generated at a relatively shallow depth. In a more recent paper [10] we presented a better model calculation showing that the energy is being generated in a thin layer at about 35 kms below the surface. This depth is shallow enough for there to be sufficient water having been either leaked-in or entrained and for there to be sufficient carbon available as well. Furthermore, this paper suggests that no deep-interior source is required for energy generation, an often argued concept in geophysics.

Toward Tresino Reactors
It should be clear that access to tresino-formation generating power might be achieved by constructing the configurations similar to those described above in the geophysical arena; namely a source of hydronium ions (water) and a source of superconducting material such as processed carbon powder as discussed by Scheike et al. [7] possibly processed at somewhat elevated temperature and pressure. If this picture is correct, experiments along these lines should reveal operating conditions for tresino-generated power reactors.
In this narrative, I have suggested how the geophysics of energy generation can be a guide to develop tresino reactors here on the surface not just at 35 kms below the surface. Of course, this will require substantial experimental efforts to be tested and perhaps then realized.
Acknowledgement
With deep gratitude, I acknowledge my late mentor, colleague and friend, Dr. John R. Reitz, without whom this work would never have become possible.
References
- Mayer FJ, Reitz JR. Electromagnetic Composites at the Compton Scale. Int J Theor Phys. 2012; 51: 322-330. doi: 10.1007/s10773-011-0959-8
- Mayer FJ, Reitz JR. Thermal energy generation in the earth. Nonlinear Process Geophys. 2014; 21: 367-378 doi: 10.5194/npg-21-367-2014
- Evans RL. Earthʼs electromagnetic environment – 3A. Conductivity of Earth Materials. In: Chave AD, Jones AG. The Magnetotelluric Method: Theory and Practice. Chapter 3. Cambridge University Press. 2012.
- Mayer FJ. Brief communication: Electron pair donors and Earths energy generation. Nonlinear Process Geophys. 2018. doi: 10.5194/npg-2018-13
- Yankowitz M, Chen S, Polshyn H, et al. Tuning superconductivity in twisted bilayer graphene. Science. 2019; 363(6431): 1059-1064. doi: 10.1126/science.aav1910
- Scheike T, Bohlmann W, Esquinazi P, Barzola-Quiquia J, Ballestar A, Setzer A. Can doping graphite trigger room temperature superconductivity? Evidence for granular high-temperature superconductivity in water-treated graphite powder. Adv Mater. 2012; 24(43): 5826-5831. doi: 10.1002/adma.201202219
- Mayer FJ. Superconductivity and low-energy nuclear reactions. Results Phys. 2019; 12: 2075- 2077. doi: 10.1016/j.rinp.2019.02.027
- Bedrosian PA, Peacock JR, Bowles-Martinez E, Schultz A, Hill GJ. Crustal inheritance and a top-down control on arc magmatism at Mount St Helens. Nat Geosci. 2018; 11(11): 865-870. doi: 10.1038/s41561-018-0217-2
- Wikipedia. Carbonatite. 2020.
- Mayer FJ, Reitz JR. A parametric heat flow model in the spherical earth. Solid Earth Sciences. 2019; 4(3): 125-127. doi: 10.1016/j.sesci.2019.07.001