Research Article
Hyaluronic Acid: Evaluation of Efficacy with Different Molecular Weights
Department for Life Quality Studies, University of Bologna, Italy
*Corresponding author: Antonio Mazzucco, Adjunct Professor, Department for Life Quality Studies, University of Bologna, Italy, E-mail: antonio.mazzucco@unibo.it
Received: February 9, 2019 Accepted: February 11, 2019 Published: February 15, 2019
Citation: Mazzucco A. Hyaluronic Acid: Evaluation of Efficacy with Different Molecular Weights. Int J Chem Res. 2018; 1(1): 13-18. doi: 10.18689/ijcr-1000103
Copyright: © 2019 The Author(s). This work is licensed under a Creative Commons Attribution 4.0 International License, which permits unrestricted use, distribution, and reproduction in any medium, provided the original work is properly cited.
Abstract
Hyaluronic acids are compared to different molecular weights, initially evaluating their chemical stability and microbiological compliance and - subsequently - by means of tests in vitro, the difference in efficacy between two classes of hyaluronic acid. In particular, we will try to understand how the molecular weight can influence the activity of hyaluronic acid and its possible anti-wrinkle effect in order to obtain highly performing cosmetic products.
The affinity of the CD44 receptor in the in vitro tests represents an interesting method for the evaluation of the biological characteristics of cosmetic ingredients, in accordance with the current regulations that require cosmetic producers to verify the claimed activity.
Keywords: Hyaluronic Acid; Proteoglycans; Fibroblasts; D-glucuronic acid.
Introduction
Discovered inside a vitreous body in 1934 and then synthesized in 1964, Hyaluronic Acid (HA) is one of the main components of connective tissues. It gives the skin its particular properties of resistance and maintenance of the shape and supports the preservation of the natural degree of hydration of the skin cells; its concentration in the body tends to decrease with the advancing of age and a lack of it leads to a weakening of the skin promoting the formation of wrinkles and imperfections. Today with the NASHA (Non Animal Stabilized HA) technology, it is possible to stabilize a synthetic hyaluronic acid of non-animal origin, but bacterial, in order to obtain a product of high purity, similar to that produced by the human body, eliminating the risk of anaphylactic reactions present in other products. In particular, it is possible to obtain it conveniently from bacterial cultures of Streptococcus equis var, zooepidemicus or from recombinant strains of Bacillus subtilis by a fermentation process. This progress has led to greater availability of HA on the international market with the consequent possibility of a wider use in the cosmetic sector. Since hyaluronic acid is a natural substance that is already present in our body, once applied it is slowly and gradually reabsorbed by the body itself.
Hyaluronic Acid
Hyaluronic acid is a complex carbohydrate, belonging to the category of mucopolysaccharides, more modernly called glycosaminoglycans (GAGs). Unlike all the other compounds of the same class, which possess sulphate groups, they are of limited size and form glycoprotein complexes (proteoglycans); hyaluronic acid contains no sulfur, does not have a polypeptide component and reaches very high molecular weights. It is therefore a non-sulphured glycosaminoglycan with no protein core, from the non-branched polysaccharide chain produced by the aggregation of thousands of disaccharide units (Figure 1) formed in turn by D-glucuronic acid and N- acetyl-Dglucosamine combined together through alternating glycosidic bonds (β1→4, β1→3 glycosidic bonds as well as intramolecular hydrogen bonds that stabilize their conformation) [1].
In the beta configuration it is admissible for all of its cumbersome groups to be in an equatorial position (sterically favorable), while all hydrogen atoms occupy small sterically less favorable axial positions, which makes the structure of the disaccharide energetically very stable.
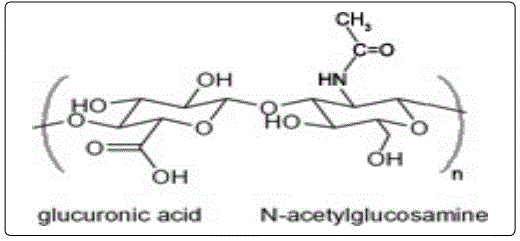
D-Glucuronic acid is an organic compound belonging to the class of alduronic acids; it derives from the carboxyl group oxidation of the primary alcohol group (OH bonded to C6) of D-glucose. N- acetylglucosamine is a monosaccharide deriving from the modification of 2-glucosamine which undergoes an N-acetylation reaction. These substances are both negatively charged and when they are joined together, the strong repulsion gives rise to a linear, flexible and extremely polar molecule [2].
In aqueous solution, the polyelectrolyte character of the macromolecule (mutual repulsions of the negative charges on the carboxyl groups) and the intra-molecular hydrogen bonds cause the HA to appear as a ball and occupy a high hydrodynamic volume, whose size depends on the concentration of the polymer and the presence of fillers in the solvent.
At a physiological pH the carboxyl groups of the glucuronic units are therefore ionized, giving the hyaluronate molecule a high polarity, and consequently a high solubility in water. Thanks to this property, hyaluronate is able to mix with many molecules of water reaching a high degree of hydration [3].
In addition to these properties, hyaluronic acid can also interact with membrane proteins or surface cell receptors belonging to three main classes: CD44, RHAMM and ICAM-1 [4]. The interaction with these receptors triggers cellular responses and different metabolic processes in relation to the respective molecular weights. About one third of cutaneous HA is contained in the epidermis where, by binding to the CD44 receptor (Figure 2), it ensures not only the adhesion between the individual keratinocytes, but also [5] intervenes in the regulation of gene expression [6]. It has been shown how HA has a positive effect on the proliferation of skin fibroblasts and how CD44 plays a fundamental role in this. New research has confirmed that HA is produced dynamically by most skin cells, not only by fibroblasts but also by keratinocytes in the outer protective layer (epidermis). For both fibroblasts and keratinocytes, HA plays a regulatory role in the control of cell physiology through the interaction of extracellular HA with the cell surface receptor, CD44. This interaction mediates intracellular signaling both directly and indirectly, through CD44 interactions with the cytoskeleton and with EGF receptors (Epidermal growth factor: or epidermal growth factor receptors) and TGFβ (Transforming growth factor: Transforming growth factor beta) [7].
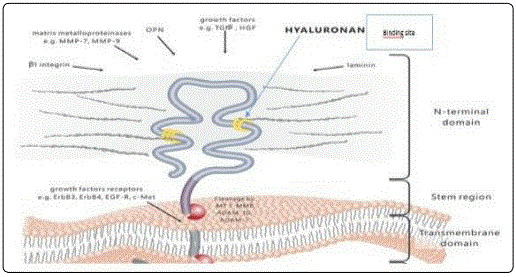
CD44 (Cluster of Differentiation 44) is a transmembrane glycoprotein of type I, monomeric and ubiquitous, encoded by a single gene and expressed in different isoforms all endowed with the interaction site for hyaluronic acid; it is mainly a molecule of intercellular adhesion localized on lymphocytes and leukocytes. The CD44 is composed of 177 AA and is divided into 4 domains among which an extracellular called HBD (Hyaluronan Binding Domain) that represents the site for [8] hyaluronic acid, a transmembrane with anchor function, an intermediate domain between the two, which varies in length according to the exons, and an intracellular one with various functions (Figure 3) [9]. The HBD domain is 157 amino acids long and has 3 disulfide bridges and numerous O- and N-glycosylation points to which up to two hundred glucidic residues of various types are linked and with various bonds (xylose, N-acetylglucosamine, galactose, etc.), many to almost completely mask the basic protein and modify its affinity for the various ligands; furthermore, every cell type produces a protein with a specific glycosylation sequence. Another important aspect that significantly modifies affinity is the possibility of having up to 10 alternative splicing, the basic protein is called CD44H (H: hematopoietic) or CD44s, while isoforms are indicated with CD44v x (where instead of x indicates one or more numbers from 1 to 10), these show lower affinity for hyaluronic acid.
The binding with this receptor does not have simple anchoring function but causes the protein to transduce signals inside the cell with variable consequences depending on the cell. The interaction between hyaluronic acid and CD44 has been implicated in a variety of physiological events, which include cell-cell adhesion, cell- substrate adhesion, migration, cell proliferation and activation, up-take and hyaluronic acid degradation. Recent studies suggest that the two main functions of CD44 in the skin are the regulation of keratinocyte proliferation in response to extracellular stimuli and the maintenance of local hyaluronic acid homeostasis [10].
Hyaluronic acid is a component of the so-called fundamental substance of the dermis and, to date is the main substance of anti-aging research thanks to its ability to hydrate, lubricate and elasticize the skin, maintain the shape of the tissues and reinforce the tone. Furthermore, the application of topical hyaluronic acid helps to support the synthesis of collagen.
Hydration, turgidity, plasticity and all the particular properties of the dermis are therefore influenced by its determining role, as well as the protection against free radicals with scavenger antioxidant action, the collagen neosynthesis and other important biological activities mainly dependent on its weight molecular. It has also been shown that the fragments of the anti-aging molecule par excellence stimulate the activity of fibroblasts, thereby favoring the synthesis of hyaluronic acid. In the epidermis, hyaluronic acid, thanks to its negative charge, is located in the extracellular region where it forms a hydrophilic network capable of transporting nutrients and metabolites to the different cell types through a percolation mechanism.
Hyaluronic acid with different molecular weights
Approximately half of our body’s hyaluronic acid is distributed in the cutaneous region where molecules of different molecular weight are synthesized by fibroblasts, keratinocytes and endothelial cells that produce hyaluronic acid families of weights between 50 kDa and 3000 kDa.
The hyaluronic acid with different molecular weights has the peculiar characteristic of channeling its intervention towards different skin levels:
a) HA with high molecular weight: ceases on the surface of the epidermis to create an invisible film coating capable of blocking outgoing water and limiting evaporation thanks to the interactions it establishes with the main molecules present in this compartment (proteoglycans, glycoproteins, elastin and seven types of collagen). This thin protective film coating helps keep the skin soft, smooth and hydrated. In practice, the topical application of this macromolecule determines the formation of an external film coating that reduces perspiratio insensibilis and improves, indirectly, skin hydration. Their size, however, does not allow a good interaction with the receptors due to the steric encumbrance (Figure 3).
b) HA with a medium molecular weight: it overcomes the skin barrier and provides the skin with all the water necessary to preserve the principal sum of beauty and turgor intact. Its dimensions are optimal to guarantee the best interactions with surface receptors (Figure 3).
c) HA with low molecular weight: it boasts the unique peculiarity of knowing how to penetrate into the epidermis until reaching the subcutaneous layers and favoring the physiological production of collagen, a protein that is responsible for preserving the density and compactness of the skin. The low molecular weight increases the possibilities of penetration through the skin ensuring a better moisturizing effect, it also determines a temporary filling of tissues and smoothing of small wrinkles. It has, however, low affinity for receptors [11].
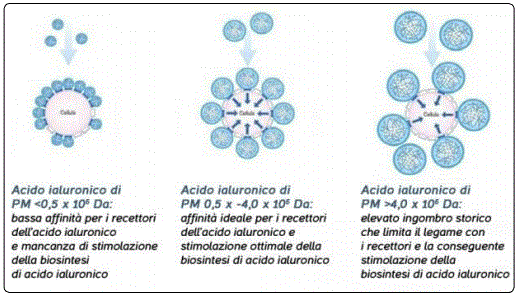
The aim of the work is to compare the effectiveness of a “standard” hyaluronic acid (1,000-1,800 kDa) compared to a so-called “second generation” hyaluronic acid, ie with a wider range of molecular weights (50-2,500 kDa). The second generation hyaluronic acids have been designed to take advantage of the moisturizing and anti-wrinkle properties; in fact, aesthetic medicine aims to exploit the nutritive-reshaping action of hyaluronic acid deriving from the synergy of the activities connected with its molecular weight.
Materials and Methods
Solutions A and B containing respectively hyaluronic acid with weight molecular ranging from 1,000- 1,800 kDa and hyaluronic acid with weight molecular comprised between 50-2,500 kDa were prepared according to the GLP (Good Laboratory Practice) as required by European Regulation No. 1223 of 2009. The solutions (sol A and sol B) are exactly the same (Table 1). In this regard, aqueous solutions for external use containing the two different hyaluronic acids have been prepared.
A: hyaluronic acid weighing 1,000-1,800 kDa
B: hyaluronic acid weighing between 50-2,500 kDa
After verifying the stability of the following solutions and having carried out the microbiological analyses, tests were carried out in vitro in order to verify and compare their anti-wrinkle efficacy. The solutions are prepared with a cold process by combining the phase 1 components, carefully mixed with turbo emulser, to those of phase 2. The two phases are continuously stirred until complete mixing. Attention must be paid to the solubility of hyaluronic acid, which has a solubilization rate dependent on its molecular weight; the higher molecular weight molecules dissolve slower. In the case of solution B, in fact, having hyaluronic acid with a molecular weight up to 2,500 kDa, it was dissolved in glycerol and then joined to the aqueous phase where sodium benzoate and tetracylic ethylenediamine acid had previously been solubilized. In the case of solution A, instead, hyaluronic acid is directly dissolved in demineralized water together with the other components of step 1.
The entire dissolution process is facilitated by the use of a benchtop turboemulser, which allows reducin g considerably the time that would be required for normal stirring. Finally, the pH is measured which must be included in the range 4.5-5.5 and optionally corrected with citric acid.
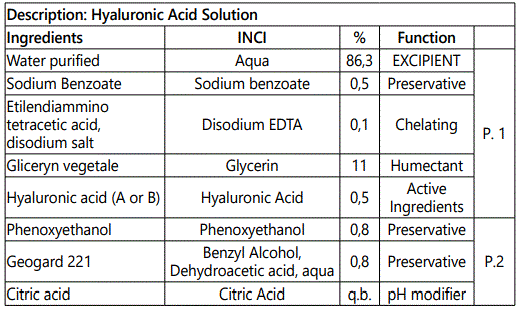
As required by the European Regulation n. 1223/2009, “cosmetic products made available on the market must be safe for human health when used under normal or reasonably foreseeable conditions of use”. This Regulation prohibits experimentation on animals both of finished cosmetic products and of ingredients (or combinations of ingredients) intended to be contained in cosmetic products. This restriction has favored the development of alternative methods in vitro to protect companies and consumers and that, unlike the animal model, allow the experiment to be carried out without numerical limits and under conditions of greater control and standardization, as well as to apply quantitative and objective measurement systems. In addition to being an alternative to animal testing, as they lack prejudicial ethics and potentially quicker and less expensive, tests in vitro have other advantages; in particular [11], allow the use of normal human cells and precise control of the doses of the substances used.
Tests in vitro are generally carried out to highlight the performances provided by ingredients or finished products but also to be able to be compared and quantified and, for this, must be reliable and reproducible. These tests can therefore be used as screening tools during the development of the product but also to illustrate the mode of action of the components. After verifying the stability of the solutions and compliance with microbiological analyses, Tests in vitro were carried out in order to evaluate their efficacy and safety. These tests are carried out on specific skin cell lines by professionals in the sector at specialized laboratories and university facilities.
Cytotoxicity test
As far as Solutions A and B are concerned, a preliminary evaluation was carried out through in vitro cytotoxicity tests on cellulary cultures of fibroblasts. This test allows to evaluate in vitro the ability of an agent to inhibit cell growth. The MTT assay was used to determine the possible cytotoxicity of Solutions A and B. The cell viability test (or MTT assay) is a colorimetric test (Figure 4) that allows estimating the number of live cells present in culture and therefore evaluating the effect of treatment with an external agent on the viability of the cell population.
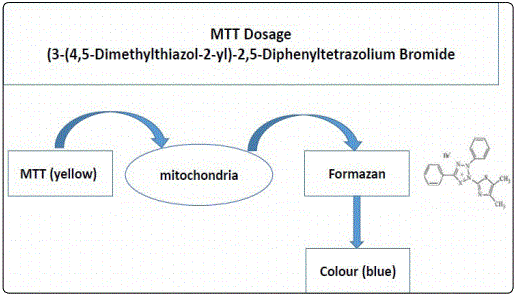
The average cell viability at different concentrations was expressed as a percentage relative to the mean value of the negative controls. A reduction in cell viability greater than 30% is considered a cytotoxic effect.
Results
The samples Sol A and Sol B at 3 different concentrations (0.62; 0.31; 0.16) under the test conditions described herein n or n cause cytotoxic effects with an IC50 of 3.92 mg/ml (Table 2). All the criteria defined for the checks for the acceptability of the test are respected. The standard deviation among the six replicates of the samples is well within the defined limits of 18%. After verifying that Solutions A and B do not give cytotoxicity problems, a quantitative analysis was performed using Western Blot to determine the presence of CD44.
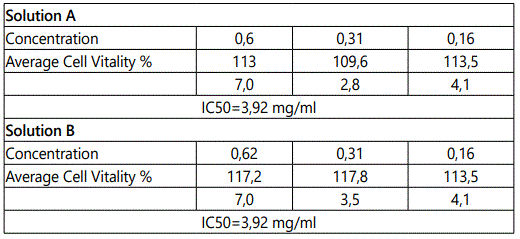
Western blot quantitative analysis
As previously noted CD44 is the major cell surface receptor for hyaluronic acid and is expressed in many cell types including fibroblasts. The terminal amino group shares the homologous sequence with hyaluronans.
The cell extracts were then treated with solutions A and B and western blot was used to highlight changes in protein levels of CD44. Western blot is a biochemical technique that allows to identify a particular protein in a mixture of proteins, through the recognition of specific antibodies; in general, to facilitate recognition, the protein mixture is first separated based on their size using a polyacrylamide gel; subsequently, the proteins are transferred onto a support, which is commonly a nitrocellulose membrane, and then the protein is recognized by using a specific antibody.
The cells were first sown and incubated. Subsequently, the cell monolayers were detached from incubation culture plates with 16 mmol of EDTA in PBS for 20 minutes at 37°C and centrifuged for 10 minutes at 1000 rpm. After centrifugation, the precipitate containing the cells was resuspended in a suitable volume of lysis buffer (300 mM NaCl, 50 mM Tris HCl pH 7-6, 0/5% Triton X-100, 2 mM of phenyl methylsulfonylfluoride) for 45 minutes in ice. The cells were lysed and the protein lysate was recovered by centrifugation. 10% sodium thiosulfate and 10% sodium dodecyl sulfate were added to the supernatant up to a final concentration of 0.2%. The supernatant was dosed into its protein content through the analysis of the bicinconic acid (BCA) (Pierce, United Kingdom). The protein dosage by means of the Bicynonic Acid (BCA) assay allows to determine the total protein quantity by means of a colorimetric reaction which involves the reduction of Cu2+ copper by proteins which produce an alkaline intermediate containing the Cu1+ ion to which the Bicinconic acid (BCA, Pierce). Following the chelation of a Cu1+ ion by two molecules of BCA a violetcolored water-soluble complex is formed. The absorbance at 570 nm is then measured, which is proportional to the protein concentration.
Samples of supernatant with balanced amounts of protein for each sample were transferred to 5-7% polyacrylamide gel in non-reducing conditions; this allows separating proteins on the basis of their mass differences by electrophoresis. The separated proteins were then transferred onto a nitrocellulose membrane. Immunolabeling was performed with a CD44 antibody using Mabs F. 10.44.2, F. 10.62.1 and A3D8. The secondary antibody was a goat anti-mouse Ig conjugated with peroxidase (Southern Biotechnology, USA). The antigen was visualized by chemiluminescence by first applying the chemiluminescent reagent (ECL) and then an autoradiographic plate (Sigma, UK). The intensity of staining of the CD44 bands was measured by densitometry. The bands were scanned using a Hewlett Packard Scanjet 2C scanner connected to an Apple Macintosh IIfx with NIH Image software with gel plotting macros.
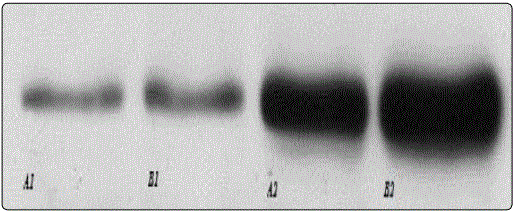
The bands represent the expression of CD44 before and after exposure with solutions A and B. As can be seen from the image (Figure 5), the band B2 is the most extensive. Thus, a higher expression of CD44 can be correlated in cells treated with solution B, i.e. with hyaluronic acid of molecular weight between 50- 2500 kDa.
Discussion
As can be seen from the tables, Solutions A and B over time do not show significant changes. The comparison between the second generation hyaluronic acids compared to the standard ones did not show any significant differences with regard to stability tests and microbiological checks; both solutions are in fact stable over time and secure. Furthermore, they did not show cytotoxicity by the MTT assay. In contrast, quantitative analysis using Western Blot has shown substantial differences between the two solutions; from the reading of the obtained bands a greater expression of CD44 protein is visible in the tissues treated with second generation hyaluronic acid (Sol. B). The comparison between the two hyaluronic acids by means of tests in vitro thus confirms the initial hypothesis of greater anti-wrinkle efficacy of Solution B containing hyaluronic acid with a molecular weight ranging from 50-2,500 kDa. The greater efficacy of hyaluronic acid with a molecular weight between 50-2,500 kDa is an expression of the synergistic action deriving from the different dimensions of the molecules, which, when combined, create the optimal conditions for preventing and combating the aging process, guaranteeing the corrective result of the wrinkle. Therefore, the anti-wrinkle effect of solution B can be considered as resulting from the different activities of:
a) HA low PM that can penetrate deeply ensuring interaction with receptors and in particular with CD44 located on cell membranes. This interaction induces a reactivation of cellular metabolism to help fight environmental stress through mitosis and cell proliferation.
b) It has a medium PM that penetrates through the skin and provides the water necessary to preserve turgidity and firmness of the skin.
c) HA with high PM that ceases on the surface of the epidermis and forms an invisible film coating capable of blocking the evaporation of water, thus counteracting dehydration.
Conclusion
The use of a second generation hyaluronic acid showed a higher affinity with the CD44 receptor. The interaction with these receptors trigger a better cellular response and different metabolic process in relation to the respective molecular weights. The in vitro test showed that although, one third of cutaneous HA is contained in the epidermis where, by binding to the CD44 receptor, it ensures not only the adhesion between the individual keratinocytes, but also intervenes in the regulation of gene expression, the II Generation Hyaluronic Acid plays an important and better role than single hyaluronic acids at different molecular weights both at the fibroblast and keratinocyte levels.
Although a higher expression of CD44 in tissues treated with second generation hyaluronic acids has been shown by Western Blot, this should be further verified with tests in vitro and, possibly, with tests in vivo that can confirm the greater anti-wrinkle efficacy of hyaluronic acid. weight between 5-2,500 kDa. It must also be considered that products containing HA are in continuous development; as proof of this, cosmetics with cross-linked hyaluronic acid have recently been marketed. The use of hyaluronic acid in the dermocosmetic field is acquiring growing interest as a valid alternative to the much more invasive techniques of aesthetic medicine.
Role of Sponsor
The funding organisations had no role in the design, collection, management, analysis and interpretation of the data; preparation, review or approval of the manuscript; and decision to submit the manuscript for publication.
References
- Olczyk P, Komosińska-Vassev K, Winsz-Szczotka K, Kuźnik- Trocha K, Olczyk K. Hyaluronan: Structure, metabolism, functions, and role in wound healing. Postepy Hig Med Dosw (Online). 2008; 62: 651-659.
- Kogan G, Soltés L, Stern R, Gemeiner P. Hyaluronic acid: a natural biopolymer with a broad range of biomedical and industrial applications. Biotechnol Lett. 2007; 29(1): 17-25. doi: 10.1007/s10529-006-9219-z
- Baumann L. Skin ageing and its treatment. J Pathol. 2007; 211(2): 241-251. doi: 10.1002/path.2098
- Turley EA, Noble PW, Bourguignon LY. Signaling Properties of Hyaluronan Receptors. J Biol Chem. 2001; 277(7): 4589-4592. doi: 10.1074/jbc.R100038200
- Csoka AB, Frost GI, Stern R. The six hyaluronidase-like genes in the human and mouse genomes. Matrix Biol. 2001; 20(8): 499-508.
- Toole BP. Hyaluronan and its binding proteins, the hyaladerins. Curr Opin Cell Biol. 1990; 2(5): 839-844.
- Samuel SK, Hurta RA, Spearman MA, Wright JA, Turley EA, Greenberg AH. TGF-beta 1 stimulation of cell locomotion utilizes the hyaluronan receptor RHAMM and hyaluronan. J Cell Biol. 1993; 123(3): 749-758.
- Juhlin L. Hyaluronan in skin. J Intern Med. 1997; 242(1): 61-66.
- Naor D, Sionov RV, Ish-Shalom D. CD44: structure, function, and association with the malignant process. Adv Cancer Res. 1997; 71: 241-319.
- Kaya G, Rodriguez I, Jorcano JL, Vassalli P, Stamenkovic I. Selective suppression of CD44 in keratinocytes of mice bearing an antisense CD44 transgenedriven by a tissue-specific promoter disrupts hyaluronate metabolism in the skin and impairs keratinocyte proliferation. Genes Dev. 1997; 11(8): 996-1007.
- Wolny PM, Banerji S, Gounou C, et al. Analysis of CD44- hyaluronan interactions in an artificial membrane system: insights into the distinct binding properties of high and low molecular weight hyaluronan. J Biol Chem. 2010; 285(39): 30170-30180. doi: 10.1074/jbc.M110.137562