Mini-Review
A Brief Review on Nanocomposites based on PVDF with Nanostructured TiO2 as Filler
1National Centre for Physics, Islamabad, Pakistan
2Department of Chemistry, Quaid-i-Azam University, Islamabad, Pakistan
*Corresponding author: Sara Qaisar, Department of Chemistry, Quaid-i-Azam University, Islamabad, Pakistan, E-mail: sara.qaisar@ncp.edu.pk
Received: September 29, 2016 Accepted: October 26, 2016 Published: November 1, 2016
Citation: Razzaq H, Nawaz H, Siddiq A, Siddiq M, Qaisara S. A Brief Review on Nanocomposites based on PVDF with Nanostructured TiO2 as Filler. Madridge J Nanotechnol Nanosci. 2016; 1(1): 22-28. doi: 10.18689/mjnn-1000107
Copyright: © 2016 The Author(s). This work is licensed under a Creative Commons Attribution 4.0 International License, which permits unrestricted use, distribution, and reproduction in any medium, provided the original work is properly cited.
Abstract
Nanocomposites are based on a combination of two or more nanomaterials. Depending on the selection of the constituents forming the composite, the end properties may be tuned as per requirements. Moreover, the combination of organic and inorganic materials can help improve the composite properties a step further, and thus provide exceptional functionalities. Owing to their exceptional mechanical and thermal stability, titania (TiO2) nanostructures are one of the most commonly used reinforcements. Thus, considerable research has focused on the development and characterization of TiO2 reinforced composites based on several different polymer matrices. Amongst the matrix materials used in different nanocomposites, Polyvinylidene Fluoride (PVDF) is the choicest matrix because of its stability, ease of processing and good mechanical properties relative to other thermoplastic polymers. This review focuses on recent progress in the incorporation of TiO2 nanostructures into a PVDF matrix so as to develop nanocomposites having outstanding optical, mechanical and electrical properties. A summary of the diverse potential applications of the PVDF/TiO2 nanocomposites has also been given.
Keywords: Nanocomposites; Polymers; Inorganic nanoparticles; PVDF; TiO2.
Introduction
Small clusters of atoms from 1 to 100 nanometers long fall under the category of nanoparticles and may be compared to smaller visible living creature - an ant which is millions of nanometers. They are not conformed to absolute quantum chemistry or laws of classical physics and hold strikingly exciting properties in comparison to their bulk counterpart due to increased surface area and quantum confinement effects leading to greater chemical reactivity and enhanced strength [1-5]. Quantum effects become much more important in determining the resultant properties of nanomaterials in terms of unusual electrical, magnetic and optical characteristics [6-8]. Nanoparticles play vital role in broad range of fields for instance pharmaceuticals, electronics and environmental remediation [9-10].
Nanoparticles may be identified as nanocomposites, aerosols, nanopowders, nanoceramics, and colloids contingent to their use of interest. These can include carbon based nanomaterials e.g., fullerenes, nanodiamonds, carbon nanotubes, graphene, metal clusters (Pt, Pd, Au, Ag) and metal oxide nanoparticles like TiO2, ZnO, Al2O3 and SiO2 biomaterials or organic materials [11]. Out of these different types of nanomaterials, metal oxide nanoparticles have been extensively explored as they are already being utilized in many commercial processes and products. They are widely employed not only as everyday items including paints and varnishes, sunscreens, cosmetics, stain-resistant clothing, sporting goods and electronics but also find applications in the field of nanomedicine as diagnostic, drug delivery and imaging agent [12-15]. Titanium dioxide nanoparticles (TiO2 NPs) have received utmost interest among other metal oxide NPs owing to its exciting properties for instance cost effectiveness, stability, commercial handiness, exceptional photocatalytic, UV-cleaning, antibacterial properties and biocompatibility [14].
Nano TiO2 usually exists in different morphological forms for instance either as spherical NPs or as nanotubes. Siegel [16] classified nanostructured materials in general as Zero dimensional (clusters), one dimensional (nanotubes), two dimensional (films) and three dimensional (polycrystalline) nanostructures as represented in figure 1.

Properties of the nanoparticles can be exploited for their utilization in various exciting future technologies by controlling their morphology. For instance, nanotubes are preferred in applications including need to create electrical bridges in conducting materials or to store/ process information in magnetic devices [17].
Features of TiO2- NPs vs Nanotubes
Critical properties of TiO2 which should be considered for its specific application include geometry, morphology and microstructure. These properties are summarized in Table 1.


Nanocomposite of PVDF with TiO2 nanostructures as nanofiller and applications
Nanoscale innovative material design and synthesis holding unusual properties is important in fabrication of advanced devices for optics, electronics and biotechnology. In this perspective, polymers are considered as a usual choice based on their high surface area and low cost. However the polymeric material lack in mechanical and thermal stability. Thus, in polymer science, there is a dire need to widen the application window by enhancing their mechanical, thermal, and electrical properties. Among the polymeric material, Polyvinylidene fluoride (PVDF) is a semi crystalline polymer posses incredible properties, such as excellent resistance to chemicals, good thermal stability, high mechanical strength and inflammable, etc. Figures 2 and 3 PVDF has been extensively employed in various fields, such as nuclear-waste processing, waste water treatment, pulp and paper industry, chemical processing industry and as piezoelectric material [24-26]. Reinforcement of polymers with incorporation of whisker, platelets, fibers or nanoparticles may be considered as analternative approach to attain improved properties in nanocomposites [27]. Polymer nanocomposites have shown promising ability to maintain balance among customary properties including ease of fabrication and low weight of polymers with the toughness and strength of reinforcing material [28]. TiO2 is extensively used as filler material for PVDF matrix owing to its promising role in enhancement of mechanical, thermal, electrical and optical properties of PVDF/TiO2 nanocomposite for potential applications in variety of fields [29-30].
Nanocomposites of PVDF with inclusion of different contents of TiO2 nanofiller along with their synthesis protocol and applications have been collected in table 1.


L.Yu et al., reported 30% enhancement in tensile strength of PVDF/TiO2 hollow fibre membranes prepared via blending or method sol-gel [49]. Similarly, an improvement in the tensile strength of PVDF hollow fibre membranes from 1.71 MPa to 3.74 MPa was observed upon incorporation of a combination of 1 wt % Al2O3 and 2 wt % TiO2 in PVDF matrix. The authors attributed this improvement to the formation of compact macro void in the nanocomposite [50]. There is considerable impact of addition TiO2 on the thermal and electrical properties of PVDF/ HfP as reported by K. Prabakaran et al [51]. They reported about two fold enhancement in the dielectric constant of nanocomposite upon inclusion of surface modified TiO2 NPs. Moreover, significant changes in optical properties of PVDF could be observed by incorporation of TiO2.
NPs e.g., Zhang et al and A. Munoz-Bonilla et al have reported the transmittance spectra of PVDF/ ZrO2-TiO2 and PVDF/TiO2 nanocomposite [52-53]. They showed an increase in the absorption and refractive index of the nanocomposite in the presence of TiO2 NPs. In fact, enhancement in different properties of PVDF/TiO2 nanocomposite is attributed to the interaction between the filler material and polymer matrix. It has been reported that the fluorine atom of the PVDF polymer can interact strongly with Ti4+ ions via Vander Waal forces of interaction to induce mechanical stability to nanocomposites for various exciting applications as shown pictorially in figure 4 [54].

The cumulative effect of both PVDF and TiO2 in nanocomposites is more effective than the individual components. Different combinations of nanocomposites including some other polymer or nanomaterials in the matrix of PVDF have been explored for multiple purposes in various fields of research. Hence, in the following section, different applications of PVDF/ TiO2 nanocomposites are briefly reviewed (fig 5).
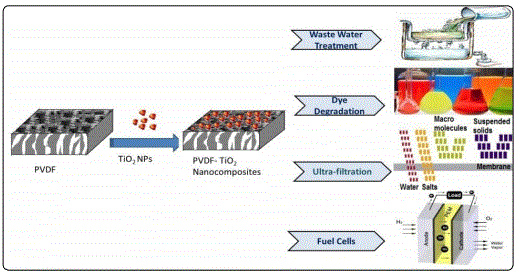
Environmental application
Extensive reports are available in literature for the utilization of PVDF/ TiO2 nanocomposite as water treatment membranes, removal of heavy metals and photocatalytic degradation of dyes in textile industry [55]. TiO2 nanofiller attributes to the enhancement of hydrophilic and antifouling properties of PVDF due to the presence of OH surface groups [56-63]. G. Zeng et al fabricated nanocomposite membranes by blending different contents of titanium dioxide-halloysite nanotubes (TiO2-HNTs) with PVDF matrix. The contact angle (CA) tests indicated that the hydrophilicity of membranes was significantly increased with the addition of TiO2- HNTs. The pure water flux of 3%TiO2-HNTs/ PVDF was increased by 264.8% and 35.6%, respectively, compared with pristine PVDF membrane and 3% TiO2/ PVDF membrane. An excellent anti-fouling performance exhibited by TiO2-HNTs/PVDF membrane was attributed to hydrophilic nanoparticles which resisted the hydrophobic contaminants in waste water [64]. Safarpour M et al. [43] reported rGO/ TiO2 blended PVDF membrane prepared by the phase inversion method. The hydrophilicity and permeability of the blended membranes were enhanced due to the addition of the rGO/ TiO2 nanocomposite containing various oxygenated hydrophilic groups. Also, the rGO/ TiO2 blended PVDF membranes had higher flux recovery ratios compared with the bare PVDF membrane. On the basis of the achieved hydrophilicity, pure water flux, and antifouling results, the best content of rGO/ TiO2 nanocomposite was 0.05 wt % in the casting solution. Hence, rGO/ TiO2 nanocomposite is an excellent antifouling additive having promising applications in the membrane field [43]. In another study it is demonstrated that PVDF/TiO2 nanocomposite membranes displayed outstanding permeability performance tests even higher than commercially available PVDF membrane due to the extraordinary pore connectivity between the filler material and matrix [60]. Incorporation of TiO2 also enhanced the protein resistance of membranes significantly due to hydrophilic nature of the NPs [60]. Nanocomposite membranes based on PVDF/ TiO2 have been reported by different researchers for the degradation of dyes owing to photocatalytic effect of TiO2 NPs. N.A.M. Nor et al., recently reported nanocomposite membrane of PVDF/TiO2 for decomposition of organic pollutant from waste water [45]. H.P. Ngang et al developed PVDF–TiO2 mixed-matrix ultrafiltration membrane for the degradation of methylene blue (MB). Although, the performance of the nancomposite membrane was little affected at higher concentration of MB yet it exceeded the neat PVDF membrane due to the extra adsorption sites provided by TiO2 NPs for MB dye [37].
Energy
Much attention is paid to develop solid state lithium ions battery in order to overcome the issues like leakage of solution electrolyte, longer life cycle, high energy state density, electrochemical stability and mechanical strength [63]. Highly electronegative fluorine in the backbone of PVDF and its high dielectric constant make it a perfect choice as polymer matrix to be used as solid polymer electrolyte (SPE).Y-J. Wang et al reported SPE based on PVDF/ LiClO4/ TiO2 with enhanced conductivity upon inclusion of up to 10 wt% addition of TiO2 nanoparticles [34].
Another group reported lithium bis (oxalato) borate (LiBOB) based PVDF/PVC composite polymer electrolyte (CPE) membrane with 2.5wt% TiO2 exhibiting comparatively high ambient-temperature conductivity than ZrO2 with even porous network to accommodate liquid electrolyte. Mobility of Li+ ions could be facilitated due to the amorphicity of the highly conductive CPE having potential to be useful as lithium-ion electrolyte batteries [64]. Similarly, electrolyte membrane based on PEO/PVDF/TiO2 was fabricated by H. Han et al. They applied it for solid state dye-sensitized nanocrystalline solar cells. Introduction of TiO2 and electronegative fluorine of PVDF resulted in outstanding performance of the solar cell due to high conductivity and reduced recombination rate at the interface of solid state electrolyte and TiO2 NPs [65].
Electronics
Development of high energy density polymer-based dielectric materials has become a challenging topic of research interest [63]. Polymer matrices containing homogeneous Dispersion of metal oxide nanoparticles are of particular attention because of their potentially high breakdown strength and high dielectric permittivity. Such combinations may be used as an alternative approach to develop dielectric materials for energy storage and sensing applications. K. Prabakaran et al., reported PVDF/ HFP/ TiO2 nanocomposite with two fold enhancement in dielectric constant upon incorporation of TiO2 NPs [51]. In another study conducted by J. Iwagoshi et al., it is demonstrated that incorporation of rutile TiO2 NPs into PVDF host shown promising results for their use as capacitor due to enhancement in electrical properties of the nanocomposite [66]. W. L. Ong et al focused on the development of a hybrid organic–inorganic TiO2 nanocomposite, which were used as the volatile organic compound (VOC) sensing and photocatalytic degradation with production of hydrogen gas. These properties were enhanced by the well-structured TiO2 nanotubes, metal nanoparticles and reduced graphene oxide loading for enhanced light absorption and charge-transfer kinetics. Crosslinking networks were induced due to functionalization of TiO2 nanocomposite with a polyvinylidene fluoride (PVDF) matrix which produced the mechanical reinforcement-flexibility [67]. Anjum Qureshi et al investigated ac/dc electrical properties of (lithium and titanium doped nickel oxide) LTNO/ PVDF nanocomposites film as a function of temperature and as NH3 gas sensor. It was observed that electrical conductivity obeys the power law. Spin coating method was used to fabricate LTNO/ PVDF nanocomposite which was used for the sensing of ammonia gas [68].
Meng-Fang Lin et al reported PVDF-g-HEMA [poly (vinylidene fluoride)-graft-poly (2- hydroxyethylmethacrylate)/Barium Titanate (BaTiO3) nanocomposites for high energy density capacitor materials. Highest dielectric constant up to 333 was achieved [69].
Haixiong Tanget at fabricated barium titanate (BaTiO3) nanowires (NWs) in a poly (vinylidene fluoride-trifluoro ethylene-chloro fluoro ethylene) (PVDF-TrFE-CFE)) matrix. High breakdown strength and high dielectric permittivity were reported for these nanocomposites [70]. Lyly Nyl Ismail et al reported the dielectric properties of multilayer PVDF-TrFE/ PMMA:TiO2 thin film. Spin coating method was used to fabricate these films .Due to the high dielectric properties, of these films are found to have wide applications in electronics industry [71].
Conclusion
In this mini review article we have discussed the morphology of TiO2 nanostructures. It is shown that 1-D TiO2 nanostructures have high aspect ratio having quite high efficiency in certain applications as for instance photocatalytic activity of TNTs is found to be 10 folds that of TNPs [23]. Apart from this, nanocomposites based on PVDF and TiO2 demonstrate versatile applications especially in the field of environmental remediation, dielectric materials and solid state polymer electrolytes. There is still room to further explore the effect of TiO2 doped with different metals, metal oxides and carbon nanomaterials to be used as filler materials for PVDF matrix. Also, little information is available in literature on the nanocomposites of TNTs with PVDF. TNTs can be more effective than TiO2 nanoparticles due to the ordered structure and hence can open gate for exciting application.
References
- Yang HG, Sun CH, Qiao SZ. Anatase TiO2 single crystals with a large percentage of reactive facets. Nature. 2008; 453: 638–641. doi: 10.1038/nature06964
- Chen X, Mao SS. Titanium dioxide nanomaterials: Synthesis, properties, modifications, and applications. Chemical Reviews. 2007; 107(7): 2891–2959. doi: 10.1021/cr0500535
- Hashimoto K, Irie H, Fujishima A. TiO2 photocatalysis: a historical overview and future prospects. Japanese journal of applied physics. 2005; 44(12): 8269–8285. doi: 10.1143/JJAP.44.8269
- Fujishima A, Rao TN, Tryk DA. Titanium dioxide photocatalysis. Journal of photochemistry and photobiology C: Photochemistry Reviews. 2000; 1(1): 1–21. doi: 10.1016/S1389-5567(00)00002-2
- Nowotny MK, Bogdanoff P, Dittrich T, Fiechter S, Fujishima A, Tributsch H. Observations of p-type semiconductivity in titanium dioxide at room temperature. Material Letters. 2010; 64(8): 928–930. doi: 10.1016/j.matlet.2010.01.061
- Carp O, Huisman CL, Reller A. Photo induced reactivity of titatnium dioxide. Progress in Solid State Chemistry. 2004; 32(1-2): 33-117. doi: S1 389-5567(00)00002-2
- Choi SK, Kim S, Lim SK, Park H. Photocatalytic comparison of TiO2 nanoparticles and electrospun TiO2 nanofibers: effects of mesoporosity and interparticle charge transfer. Journal of Physical Chemistry C. 2010; 114(39): 16475–16480. doi: 10.1021/jp104317x
- Rezaei B, Mosaddeghi M. Applications of Titanium Dioxide Nanoparticles. Nano- Technology in Environments Conference. 2009; 1-3. doi: 10.13140/RG.2.1.2184.6006
- Khalid NR, Ahmed E, Ikram M, Phoenix DA, Elhissi A, Ahmed W, Jackson MJ. Effects of calcination on structural, photocatalytic properties of TiO2 nanopowders prepared via TiCl4 hydrolysis. Journal of materials engineering and performance. 2013;22(2): 371-375. doi: 10.1007/s11665-012-0272-6
- Hoffman MR, Martin ST, Choi W. Environmental applications of semiconductor photocatalysis. Chemical Reviews. 1995; 95(1): 69-96. doi: 0009-2665/95/0795-0069
- Sakthivel S, Shankar MV, Palanichamy M. Enhancement of photocatalytic activity by metal deposition: Characterization and photonic efficiency of Pt, Au and Pd deposited on TiO2 catalyst. Water Research. 2004; 38(13): 3001-3008. doi: 10.1016/j.watres.2004.04.046
- Burda C, Lou Y, Chen X. Enhanced nitrogen doping in TiO2 nanoparticles. Nano letters. 2003; 3(8): 1049-1051. doi: 10.1021/nl034332o
- Wang C, Ao Y, Wang P. A facile method for the preparation of titaniacoated magnetic porous silica and its photocatalytic activity under UV or visible light. Colloids and Surfaces A: Physicochemical and Engineering Aspects. 2010; 360(1): 184-189. doi: 10.1016/j.colsurfa.2010.02.030
- Nishikiori H, Qian W, El Sayed MA. Change in titania structure from amorphousness to crystalline increasing photo induced electrontransfer rate in dye-titania system. Journal Physical Chemstry C Letters. 2007; 111(26): 9008-9011. doi: 10.1021/jp072625q
- Lai TY, Lee WC. Killing of cancer cell line by photo excitation of folic acid-modified titaniumdioxide nanoparticles. Journal of Photochemistry and Photobiology A Chemistry. 2009; 204(2-3): 148-153. doi: 35400018849530.0110
- Premchand YD, Djenizian T, Vacandio F, Knauth P. Fabrication of selforganized TiO2 nanotubes from columnar titanium thin films sputtered on semiconductor surfaces. Electrochemistry Communications. 2006; 8: 1840-1844. doi: 10.1016/j.elecom.2006.08.028
- Li G, Chen L, Graham ME. A comparison of mixed phase titania photo catalysts prepared by physical and chemical methods: The importance of the solid-solid interface. Journal of Molecular Catalysis A.Chemical. 2007; 275(1-2): 30-35. doi: 10.1016/j.molcata.2007.05.017
- You X, Chen F, Zhang J. Effects of calcination on the physical and photocatalytic properties of TiO2 powders prepared by sol-gel template method. Journal Sol-Gel Science and Technology. 2005; 34(2): 181-187. doi: 10.1007/s10971-005-1358-5
- Li G, Liu ZQ, Lu J, Wang L, Zhang Z. Effect of calcination temperature on themorphology and surface properties of TiO2 nanotube arrays. Applied SurfaceScience. 2009; 255(16): 7323-7328. doi: 10.1016/j.apsusc.2009.03.097
- Wang J, Li RH, Zhang ZH. Heat treatment of nanometer anatase powder and its photocatlytic activity for degradation of acid red B dye under visible light irradiation. Inorganic Materials. 2008; 44(6): 608-614. doi: 10.1134/S0020168508060125
- Yu J, Zhao X, Zhao Q. Photocatalytic activity of nanometer TiO2 thin films prepared by the sol-gel method. Materials Chemistry and Physics. 2001; 69(1-3): 25-29. doi: 10.1016/S02540584(00)00291-1
- Hernández S, Hidalgo D, Sacco A, Chiodoni A, Lamberti A, Cauda V, Tresso E, Saracco G. Comparison of photocatalytic and transport properties of TiO2 and ZnO nanostructures for solar driven water splitting. Physics Chemistry Chemical Physics. 2015; 17(12): 7775-7786. doi: 10.1039/C4CP05857G
- Shaalan HF. Development of fouling control strategies pertinent to nanofiltration membranes. Desalination. 2002; 153: 125–131. doi: 10.1016/S0011-9164(02)01113-X
- Razmjou A, Arifin E, Dong G, Mansouri J, Chen V. Superhydrophobic modification of TiO2 nanocomposite PVDF membranes for applications in membrane distillation. Journal of Membrane Science. 2012; 415: 850–863. doi: 10.1016/j.memsci.2012.06.004
- Yuliwati E, Ismail AF. Effect of additives concentration on the surface properties and performance of PVDF ultrafiltration membranes for refinery produced wastewater treatment. Desalination. 2011; 273(1): 226–234. doi: 35400019086397.0240
- Shi F, Ma Y, Ma J, Wang P, Sun W. Preparation and characterization of PVDF/ TiO2 hybrid membranes with different dosage of nano-TiO2. Journal of Membrane Science. 2012; 389: 522–531. doi: 10.1016/j.memsci.2011.11.022
- Liu F, Hashim NA, Liu Y, Abed MRM, Li K. Progress in the production and modification of PVDF membranes. Journal of Membrane Science. 2011; 375(1): 1–27. doi: 10.1016/j.memsci.2011.03.014
- Morihama ACD, Mierzwa JC. Clay nanoparticles effects on performance and morphology of poly(vinylidene fluoride) membranes. Brazilian Journal of Chemical Engineering. 2014; 31(1): 79–93. doi: 10.1590/S0104-66322014000100009
- Yuliwati E, Ismail AF, Matsuura T, Kassim MA, Abdullah MS. Characterization of surface-modified porous PVDF hollow fibers for refinery wastewater treatment using microscopic observation. Desalination. 2011; 283: 206–213. doi: 10.1016/j.desal.2011.02.037
- Kim KM, Park NG, Ryu KS, Chang SH. Characterization of poly (vinylidenefluoride-co-hexafluoropropylene)-based polymer electrolyte filled with TiO2 nanoparticles. Polymer. 2002; 43: 3951-3957. doi: 10.1016/S0032-3861(02)00215-X
- Kim KM, Ko JM, Park NG, Ryu KS, Chang SH. Characterization of poly (vinylidenefluoride-co-hexafluoropropylene)-based polymer electrolyte filled with rutile TiO2 nanoparticles. Solid state ionics. 2003; 161(1-2): 121-131. doi: 10.1016/S0167-2738(03)00211-X
- Kim KM, Park NG, Ryu KS, Chang SH. Characteristics of PVDF- HFP/TiO2 composite membrane electrolytes prepared by phase inversion and conventional casting methods. ElectrochimicaActa. 2006; 51(26): 5636-5644. doi: 10.1016/j.electacta.2006.02.038
- Wang YJ, Kim D. Crystallinity, morphology, mechanical properties and conductivity study of in situ formed PVDF/LiClO4/TiO2 nanocomposite electrolytes. Electrochimica Acta. 2007;52:3189. doi: 10.1016/j.electacta.2006.09.070
- Miao R, Liu B, Zhu Z, Liu Y, Wang JL, Li XQ. PVDF-HFP-based porous polymer electrolyte membranes for lithium-ion batteries. J. pow.sour. 2008; 184: 420-426. doi: 10.1016/j.jpowsour.2008.03.045
- Aravindan V, Vickraman P, Krishnaraj K. Li+ ion conduction in TiO2 filled polyvinylidenefluoride-co-hexafluoropropylene based novel nanocomposite polymer electrolyte membranes with LiDFOB. Current applied Physics. 2009; 9(6): 1474-1479. doi: 10.1016/j.cap.2009.04.001
- Ngang HP, Ooi BS, Ahmad AL, Lai SO. Preparation of PVDF–TiO2 mixed-matrix membrane and its evaluation on dye adsorption and UV-cleaning properties. J.Chem.engg. 2012; 197: 359-367. doi: 10.1016/j.cej.2012.05.050
- Zhang Y, Zhang G, Liu S, Zhang C, Xu X. Naked TiO2 capsulated in nano void microcapsule of poly (vinylidene fluoride) supporter with enhanced photocatalytic activity. J.Chem.engg. 2012; 204: 217-224. doi: 10.1016/j.cej.2012.07.124
- Damodar RA, You SJ, Chou HH. Study the self cleaning, antibacterial and photocatalytic properties of TiO2 entrapped PVDF membranes. J.Haz.mat. 2009; 172(2-3): 1321-1328. doi: 10.1016/j.jhazmat.2009.07.139
- Alaoui OT, Nguyen QT, Mbareck C, Rhlalou T. Elaboration and study of poly (vinylidene fluoride) – anatase TiO2 composite membranes in photocatalytic degradation of dyes. Applied catalysis A General. 2009; 358: 13-20. doi: 10.1016/j.apcata.2009.01.032
- You SJ, Semblante GU, Lu SC, Damodar RA, Wei TC. Evaluation of the antifouling and photocatalytic properties of poly (vinylidene fluoride) plasma-grafted poly(acrylic acid) membrane with self-assembled TiO2. J haz mat. 2012; 237: 10-19. doi: 10.1016/j.jhazmat.2012.07.071
- Hou J, Dong G, Ye Y, Chen V. Enzymatic degradation of bisphenol-A with immobilized laccase on TiO2 sol–gel coated PVDF membrane. J Mem Sci. 2014; 469: 19–30. doi: 10.1016/j.memsci.2014.06.027
- Safarpour M, Khataee A, Vatanpour V. Preparation of a Novel Polyvinylidene Fluoride (PVDF) Ultrafiltration Membrane Modified with Reduced Graphene Oxide/Titaniu Dioxide (TiO2) Nanocomposite with Enhanced Hydrophilicity and Antifouling Properties. Ind. Eng. Chem. Res. 2014; 53(34): 13370-13382. doi: 10.1080/03602551003664636
- Jian L, Chilan C.The Preparation and Tribological Properties of PVDF/TiO2 Nanocomposite. Polymer-Plastics technology and engineering. 2010; 49(7): 643-647. doi: 10.1080/03602551003664636
- Nor NAM, Jaafar J, Ismail AF, Mohamed MA, Rahman MA, Othman MHD, Lau WJ, Yusof N. Preparation and performance of PVDF-based nanocomposite membrane consisting of TiO2 nanofibers for organic pollutant decomposition in wastewater under UV irradiation. Des. 2016; 391; 89-97. doi: 10.1016/j.desal.2016.01.015
- Kim BS, Lee J. Macroporous PVDF/TiO2 membranes with three-dimensionally interconnected pore structures produced by directional melt crystallization. J.Chem engg. 2016; 301:158-165. doi: 10.1016/j.cej.2016.05.003
- Putri SA, HumairoFY, Widiastuti N, Ong CS, Jaafar J, Ismail AF. PVDF/PEG/TiO2 Hollow Fiber Membrane for Lead (II)Removal. IPTEK journal of proceedings series. 2015; 1: 61-62. doi: 10.12962/j23546026.y2015i1.1038
- Ong CS, Lau WJ, Goh PS, NG BC, Ismail AF. Preparation and characterization of PVDF–PVP–TiO2 composite hollow fiber membranes for oily wastewater treatment using submerged membrane system. Desalination and water treatment. 2015; 53: 1213-1223. doi: 10.1080/19443994.2013.855679
- Yu LY, Shen HM, XuZ L. PVDF-TiO2 composite hollow fiber ultrafiltration membranes prepared by TiO2 sol-gel method and blending method. J. App.Poly. Sci. 2009; 113(3): 1763–1772. doi: 10.1002/app.29886
- OhS J, Kim NY, Lee T. Preparation and characterization of PVDF/TiO2 organicinorganic composite membranes for fouling resistance improvement. J.mem.Sci. 2009; 345: 13–20. doi: 10.1016/j.memsci.2009.08.003
- Prabakaran K, Mohanty S, Nayak SK. Influence of surface modified TiO2 nanoparticles on dielectric properties of PVdF–HFP nanocomposites. J Mater Sci: Mater Electron. 2014; 25(10): 4590–4602, . doi: 10.1007/s10854-014-2209-3
- Qing Z, Yu, Zhao LL, Xu SL, Chao Z, Ying CX, Song LX. Preparation and Characterization of Polyvinylidene Fluoride/ZrO2- TiO2 Optical Film with Wide Band Gap and High refractive index. J.inorg. mater. 2013; 28(6): 671-676. doi: 10.3724/SP.J.1077
- Bonilla AM, Kubacka A, García M, Ferrer M, García M. Cerrada ML. Visible and ultraviolet antibacterial behavior in PVDF–TiO2 nanocomposite films. J.Euro.Poly. 2015: 71: 412–422. doi: 10.1016/j.eurpolymj.2015.08.020
- Rahimpour A, Jahanshahi M, Rajaeian B, Rahimnejad M. TiO2 entrapped nano- composite PVDF/SPES membranes: preparation, characterization, antifouling and antibacterial properties, Des. 2011; 278: 343–353. doi: 10.1016/j.desal.2011.05.049
- Madaeni SS, Ghaemi N. Characterization of self-cleaning RO membranes coated with TiO2 particles under UV irradiation. J.mem.Sci. 2007; 303: 221–233. doi: 10.1016/j.memsci.2007.07.017
- Ahmad AL, Ideris N, Ooi BS, Low SC, Ismail A. Morphology and polymorph study of a polyvinylidene fluoride (PVDF) membrane for protein binding: effect of the dissolving temperature. Des. 2011; 278: 318–324. doi: 10.1016/j.desal.2011.05.046
- Bae TH, Tak TM. Effect of TiO2 nanoparticles on fouling mitigation of ultrafiltration membranes for activated sludge filtration. J.mem.Sci. 2005; 249(1-2): 1–8. doi: 10.1016/j.memsci.2004.09.008
- Natarajan K, Natarajan TS, Bajaj HC, Tayade RJ. Photocatalytic reactor based on UV- LED/TiO2 coated quartz tube for degradation of dyes. J.Chem.engg. 2011; 178: 40–49. doi: 10.1016/j.cej.2011.10.007
- Lalia BS, Kochkodan V, Hashaikeh R, Hilal N. A review on membrane fabrication: structure, properties and performance relationship. Des. 2013; 326: 77–95. doi: 10.1016/j.desal.2013.06.016
- Chin SS, Chiang K, Fane AG. The stability of polymeric membranes in a TiO2 photocatalysis process. J.mem.Sci. 2006; 275(1): 202–211. doi: 10.1016/j.memsci.2005.09.033
- Ramesh S, Ling OP. Effect of ethylene carbonate on the ionic conduction in poly(vinylidenefluoride-hexafluoropropylene) based solid polymer electrolytes. J.Polym. Chem. 2010; 1: 702-707. doi: 10.1039/B9PY00244H
- Noor MM, Buraidah MH, Yusuf SN, Majid SR. Performance of DyeSensitized Solar Cells with (PVDF-HFP)-KI-EC-PC Electrolyte and Different Dye Materials. J.int.Phot.Ener. 2011; 1-5. doi: 10.1155/2011/960487
- Aravindan V, Vickraman P. Synthesis and characterization of LiBOBbased PVDF/PVC- TiO2 composite polymer electrolytes. Poly.engg.Sci. 2009; 49(11): 2109-2115. doi: 10.1002/pen.21463
- Han BH, Liu W, Zhang J, Zhao WZ. A hybrid poly(ethylene oxide)/Poly(vinylidene fluoride)/TiO2 nanoparticle solid state redox electrolyte for dye-sensitized nanocrystalline solar cells. Advanced functional materials. 2005; 15(12):1940-1944. doi: 10.1002/adfm.200500159
- Iwagoshi J, Dillingham TR, Stufflebeam T, Ewen C. A Study of Vapor Deposited PVDF/TiO2 Nanoparticle Films by XPS. Surface Science Spectra. 2014; 21: 10-14. doi: 10.1116/11.20130902
- Ong WL, Gao M, Ho GW. Hybrid organic PVDF–inorganic M–rGO–TiO2 (M = Ag, Pt) nanocomposites for multifunctional volatile organic compound sensing and photocatalytic degradation–H2 production. Nanoscale. 2013; 5: 11283-11290. doi: 10.1039/C3NR03276K
- Qureshi A, Altindal A, Mergen A. Electrical and gas sensing properties of Li and TicodopedNiO/PVDF thin film. Sensors and Actuators. 2009; 138(1): 71-75. doi: 10.1016/j.snb.2009.01.063
- Lin MF, Lee PS. Formation of PVDF-g-HEMA/BaTiO3 nanocomposites via in situ nanoparticle synthesis for high performance capacitor applications. J. Mater. Chem A. 2013; 1: 14455-14459 doi: 10.1039/C3TA13190D
- Ismail LN, Wahid MHM, Zulkefle H, Herman SH, Mahmood MR. Dielectric Properties of PVDF-TrFE/PMMA:TiO2. Multilayer Dielectric Thin Films. Adv.Mat.Res. 2012; 576: 582-585 doi: 10.4028