Research Article
Metal Leachate from Alkaline Battery Litters: A threat to Aquatic Organisms
Department of Biotechnology, Indian Academy Degree College-Autonomous, Bengaluru, India
*Corresponding author: Sibi G, Head, Department of Biotechnology, Indian Academy Degree CollegeAutonomous, Bengaluru, India, E-mail: gsibii@gmail.com
Received: March 26, 2019 Accepted: April 1, 2019 Published: April 8, 2019
Citation: Grover S, Sibi G. Metal Leachate from Alkaline Battery Litters: A threat to Aquatic Organisms. Int J Microbiol Curr Res. 2019; 1(1): 26-28. doi: 10.18689/ijmr-1000105
Copyright: © 2019 The Author(s). This work is licensed under a Creative Commons Attribution 4.0 International License, which permits unrestricted use, distribution, and reproduction in any medium, provided the original work is properly cited.
Abstract
Disposal of spent alkaline batteries into the environment is a major concern as the metal leachate enters into aquatic environments. Microalgae are widely used in metal toxicity assay since they are sensitive organisms with a high capacity of bioaccumulation due to their high surface of contact. In this study, zinc and manganese were recovered from spent batteries through acid treatment and the leachate was tested on Chlorella vulgaris for adverse effects. Three acids namely, sulphuric, phosphoric and hydrochloric acid were used as leaching solution to recover the metals from alkaline batteries. Microalgal cells were exposed to the metal leachate and the adverse effects by means of morphological changes were observed. All the tested metal leachates have produced detrimental changes within three minutes of exposure. The results revealed the possible toxicity of metal leachate from battery litters into the environment and its detrimental effect on microalgae.
Keywords: Zinc; Manganese; Batter litter; Metal leachate; Chlorella vulgaris.
Introduction
Alkaline batteries that contain zinc and manganese dioxide due to their easy use and low cost are widely used. The chemically active components are high purity zinc powder for the anode, electrolytically produced manganese dioxide for the cathode and concentrated potassium hydroxide solution for the electrolyte. The management of spent batteries has been an issue of environmental concern. Batteries represent a large volume of toxic and hazardous materials [1]. Urban battery litter contains mainly AA and AAA size batteries accounted for more than 90% of the total [2]. Pollution caused by the alkaline batteries was less than that of zinc carbon because the former contain less lead (Pb) and cadmium (Cd) than the latter [3]. The zinc anode of dry cells contains low percentages of Cd and Pb for improvement in strength and ductility. Among the battery types used for various purposes, majority are single use batteries that find their way to landfills or incinerators.
The designs, manufacture, recycle and disposal of batteries all necessitates some form of hazardous waste management [1]. Basically battery is disposed by four methods: composting, incineration, land filling and recycling. Land filling was the most frequently used; and recycling was the most preferred by industry and environmentalists [4]. With any battery disposal method, the potential exists to release heavy metals into the environment through landfill leachate or incineration stack gases. The disposal of batteries into the environment leads to leaching of heavy metals from landfills into ground water, or volatilize into the atmosphere during combustion at open dumpsites [5].
Microalgae are widely used in metal toxicity assay since they are sensitive organisms with a high capacity of bioaccumulation due to their high surface of contact [6]. In this study, Chlorella vulgaris was used as model organism to evaluate the detrimental effect of zinc and manganese recovered from spent alkaline batteries through acid treatment. The cell morphology of microalgae exposed at various time intervals to metal solution from battery powder was considered as a parameter of metal toxicity.
Materials and Methods
Organism and growth conditions
Monoalgal, axenic culture of Chlorella vulgaris was used in this study. The algae were grown in Boldʼs basal media with continuous illumination (white fluorescent light, 132 mmol photons m-2 sec-1).
Battery dismantling and leaching process
Spent alkaline batteries of AA size were manually dismantled using a hammer mill. The other components of battery such as plastic films, ferrous scraps and paper pieces were scrapped. The black powder obtained containing zinc and manganese was used for the experiments.
Leaching
0.1 g of the dry black powder obtained was transferred to beakers containing 10 ml of leaching solution (Sulphuric acid, hydrochloric acid, phosphoric acid). A magnetic mixer with heating system was used to provide good contact between the powder and leaching solution. After the leaching period, the mixture was filtered and the filtrate was used for the experiments.
Effect of battery leachate on microalgae
The effect of zinc and manganese obtained from spent battery (leachate) was evaluated on the morphological changes of C. vulgaris. 0.1 ml of microalgal culture was added with 0.9 ml of leaching solution and the mixture was allowed to stand for 5 minutes. 2-3 drops of the treated microalgal cells were taken at every minute and observed under a microscope for morphological changes. Acid alone was used as control.
Results
The batteries used in this work were a common brand, AA size spent batteries. Acidic and alkaline solutions were tried to dissolve the battery powder along with distilled water. Among the solutions tested, acidic solution involving H2SO4 was dissolving the battery powder completely resulted in a black solution. Concentrated sulphuric acid, hydrochloric acid and phosphoric acid were used to recover zinc and manganese from the spent batteries. The recovery of both Zn and Mn from batteries treated with H2SO4 can be written according to following equations.
ZnO+H2SO4 → ZnSO4+H2O
Mn2O3+H2SO4 → MnSO4+MnO2+H2O
The effect of zinc and manganese recovered from battery powder through acid treatment was evaluated by microalgal exposure time. The detrimental effect of heavy metals from spent batteries was higher with increasing time of exposure to C. vulgaris (Figures 1-3). Among the acids tested, hydrochloric acid had more impact on the morphological changes than the sulphuric and phosphoric acid. The microalgal cells were treated with battery leachate prepared by various acid treatments and observed under a microscope for morphological changes for a period of three minutes. Above this period, most of the cells were disappeared thus indicating the lethal effect of battery leachate. In general, all the three acids were produced detrimental effect on C. vulgaris revealing the metal toxicity from spent batteries.
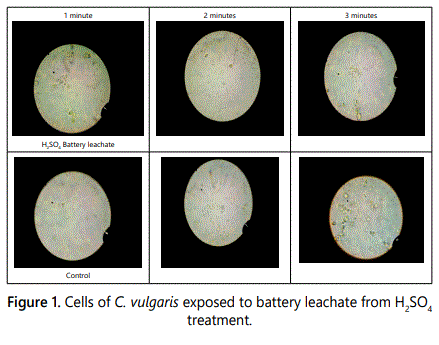
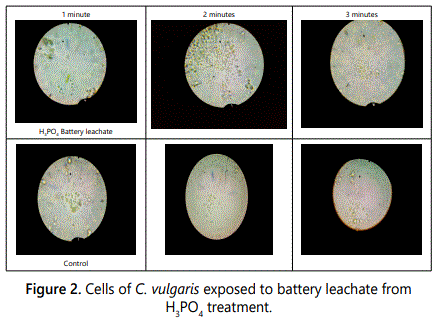
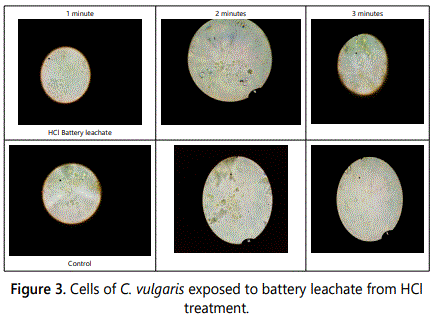
Discussion
The features of batteries make them an efficient power source. The consumption of batteries has increased because of the versatility, reduced cost and its requirements by the electronic devices. H2SO4 was used to recover the metals from alkaline batteries [7,8]. In this study, the experiments were carried on using diluted sulfuric acid in the range of 0.1 N, 0.3 N and 0.5 N as the leaching stage with these concentrations of sulfuric acid is commonly used to dissolve ZnO [9]. Spent battery electrode powder, containing Mn and Zn was treated via reductive leaching by H2SO4 and 0.5 mol/L of H2SO4 was found optimal reductive leaching condition [10].
Metal ions can enter the cells if the cell wall is disrupted by natural or artificial force [11] and compartmentalized into different sub cellular organelles. In this study, the acidic pH could have caused the cell wall damage in microalgae that facilitates the entry of zinc and manganese. Zinc is an essential trace element for aquatic organisms and humans however high concentrations of zinc may cause adverse effects to the organisms [12-15]. Zinc toxicity to the microalgae Scendesmus obliquus was reported by Li et al. [16] with LC50/EC50 value ranged between 0.057–0.217 mg/l. The effect of zinc and manganese on the microalgae Pavlova viridis was studied by Li et al. [17,18] and the increase in antioxidant enzyme activity indicated the oxidative stress caused by the metals. For zinc, growth inhibition may not be related to the intracellular metal concentration, but to extracellular zinc [19]. The possible mode of toxic action of zinc is related to the cell membrane, where it may disrupt the uptake of calcium which is necessary for the Ca-ATPase activity in cell division [20]. Manganese toxicity to terrestrial plants and humans has been reported [21,22]. Based on the previous studies, it was reported that both zinc and manganese has adverse effects on aquatic organisms. The disposal of spent batteries into the environment leads to leaching of toxic metals into terrestrial and aquatic habitats. From this study, the detrimental effect of battery leachate on C. vulgaris has proven that alkaline battery waste are of major concern in affecting the microalgae and other aquatic organisms.
Conclusion
This study investigated the toxicity of metals recovered from spent battery on the microalgae, Chlorella vulgaris. Sulphuric acid, phosphoric acid and hydrochloric acid were used as leaching solutions to recover zinc and manganese from alkaline batteries. The microalgal cells were treated with battery leachate and within 3 minutes of exposure, the cells were killed and were confirmed by the discoloration and changes in the morphological characteristics. The results revealed the toxicity of metal leachate from battery litters into the environment and its detrimental effect on microalgae.
References
- Lankey R, McMicheal F. Rechargeable battery management and recycling: Green design educational module. Green Design Initiative Technical Report, Carnegie Mellon University, Pittsburgh. 1999: 1-14.
- Jennings AA, Hise S, Kiedrowski B, Krouse C. Urban battery litter. J Environ Eng. 2009; 135(1): 46-57. doi: 10.1061/(ASCE)0733-9372(2009)135:1(46)
- Osibanjo O, Eyanohonre AE, Nnorom, IC. Heavy metals in alkaline and zinc-carbon dry cells as pollution indicators of spent batteries. European Journal of Scientific Research. 2008; 20(3): 593-603.
- Palchy J. Mineral commodity summaries-Cadmium. US Geological Survey. 1998: 1-9.
- DeLorenzo ME, Taylor LA, Lund SA, Pennington PL, Strozier ED, Fulton MH. Toxicity and bioconcentration potential of the agricultural pesticide endosulfan in phytoplankton and zooplankton. Arch Environ Contam Toxicol. 2002; 42(2): 173-181. doi: 10.1007/s00244-001-0008-3
- Ige J, Akanni MS, Morakinyo MK, Owoyomi O. A kinetic study of the leaching of iron and manganese from a Nigerian Tantalite-Columbite Ore. Journal of Applied Sciences. 2005; 5(3): 496-502. doi: 10.3923/jas.2005.496.502
- Bankole EO, Samson AE, Adebisi AS, Folawewo DA, Ogunbowale KS. Effects of Different pH Values on Dissolution and Recovery of Zinc and Manganese from Spent Zinc– Carbon Batteries Using Ascorbic Acids. Chemical Science International Journal. 2017; 21(1): 1-9. doi: 10.9734/CSJI/2017/36624
- Liddel DM. Handbook of Non-Ferrous Metallurgy: Recovery of Metals. McGraw-Hill. USA. 1945: 379-443.
- Chen WS, Liao CT, Lin KY. Recovery zinc and manganese from spent battery powder by hydrometallurgical route. Energy Procedia. 2017; 107: 167-174. doi: 10.1016/j.egypro.2016.12.162
- Wang J, Chen C. Biosorption of heavy metals by Saccharomyces cerevisiae. Biotechnol Adv. 2006; 24(5): 427-451. doi: 10.1016/j.biotechadv.2006.03.001
- Skidmore J. Toxicity of zinc compounds to aquatic animals, with special reference to fish. The Quarterly Review of Biology. 1964; 39(3): 227-248.
- Smirnova ON, Melʼnichenko EI. Study of zinc and cadmium chloride toxicity using human embryo fibroblast cultures. Gig Tr Prof Zabol. 1990; 49-50.
- Marasinghe Wadige CP, Taylor AM, Maher WA, Krikowa F. Bioavailability and toxicity of zinc from contaminated freshwater sediments: linking exposure-dose–response relationships of the freshwater bivalve Hyridella australis to zinc-spiked sediments. Aquat Toxicol. 2017; 156: 179-190. doi: 10.1016/j.aquatox.2014.08.012
- Li XF, Wang PF, Feng CL, Liu DQ, Chen JK, Wu FC. Acute toxicity and hazardous concentrations of zinc to native freshwater organisms under different pH values in China. Bull Environ Contam Toxicol. 2018. doi: 10.1007/s00128-018-2441-2
- Li M, Hu CW, Zhu Q, Chen L, Kong Z, Liu Z. Copper and zinc induction of lipid peroxidation and effects on antioxidant enzyme activities in the microalga Pavlova viridis (Prymnesiophyceae). Chemosphere. 2005; 62(4): 565-572. doi: 10.1016/j.chemosphere.2005.06.029
- Li M, Zhu Q, Hu CW, Chen L, Liu ZL, Kong ZM. Cobalt and manganese stress in the microalga Pavlova viridis (Prymnesiophyceae): effects on lipid peroxidation and antioxidant enzymes. J Environ Sci (China). 2007; 19(11): 1330-1335. doi: 10.1016/S1001-0742(07)60217-4
- Wilde KL, Stauber JL, Markich SJ, Franklin NM, Brown PL. The effect of pH on the uptake and toxicity of copper and zinc in a tropical freshwater alga (Chlorella sp.). Arch Environ Contam Toxicol. 2006; 51: 174. doi: 10.1007/s00244-004-0256-0
- Stauber JL, Florence TM. Mechanism of toxicity of zinc to the marine diatom Nitzschia closterium. Mar Biol. 1990; 105(3): 519-524. doi: 10.1007/BF01316323
- Adams F, Wear JI. Manganese toxicity and soil acidity in relation to crinkle leaf of cotton. Soil Sci. Soc. Am. J. 1957; 21(3): 305-308. doi: 10.2136/sssaj1957.03615995002100030014x
- Bader M, Dietz MC, Ihrig A, Triebig G. Biomonitoring of manganese in blood urine and axillary hair following low-dose exposure during the manufacture of dry cell batteries. Int Arch Occup Environ Health. 1999; 72(8): 521-527. doi: 10.1007/s004200050410